
Perturbations
& Behavior
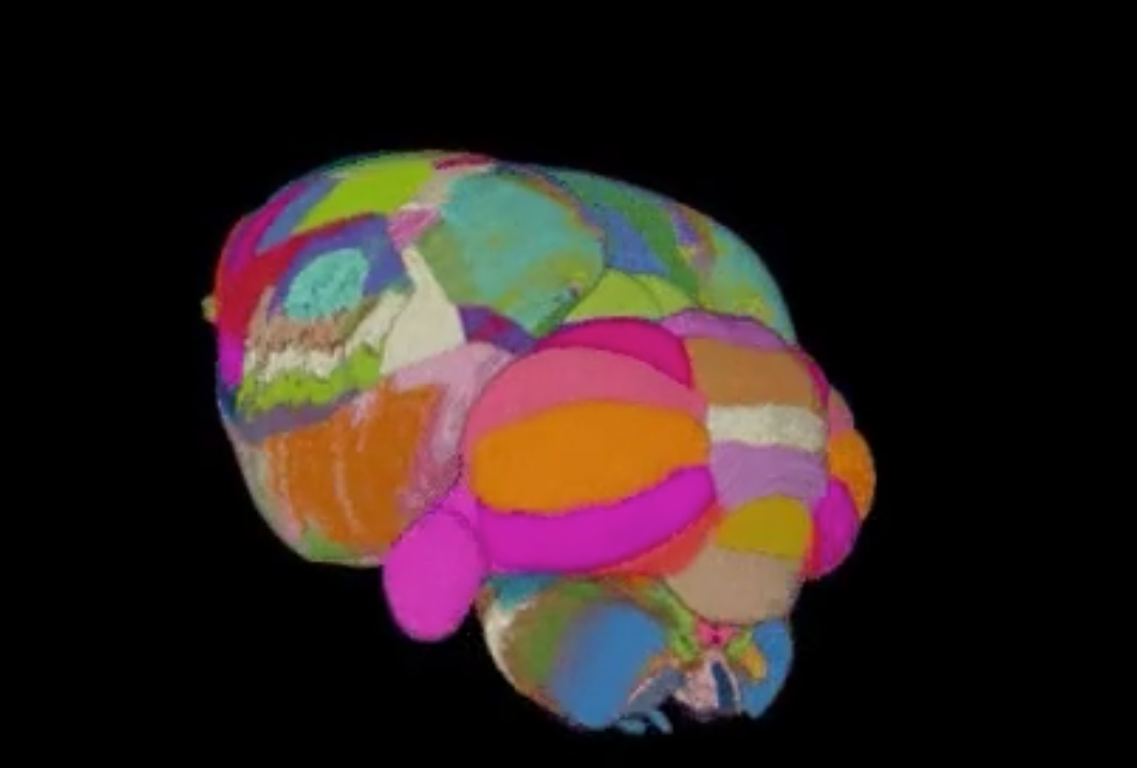
Perturbations & Behavior
Carlos Brody, Ilana Witten, Sam Wang, David Tank,
Scott Bolkan, Ben Engelhard, Esmeralda Fonseca de la Cruz, Sue Ann Koay, Edward Nieh, Michael Siniscalchi
Working memory, the ability to temporarily hold multiple pieces of information in mind for manipulation, is central to virtually all cognitive abilities. This multi-component research project aims to comprehensively dissect the neural circuit mechanisms of this ability across multiple brain areas. The individual parts of the project cohere conceptually, in part, because they all involve rodents trained to perform a type of decision-making task that is based on the gradual accumulation of sensory evidence and thus relies on working memory. Neural correlates of working memory and decision-making are distributed across a very wide range of cortical and subcortical regions, but a complete listing of which of these regions actually cause these processes—and most importantly, what the nature of their contribution might be—remains out of reach. A significant obstacle has been that these processes can evolve rapidly (for example, going from loading an item into working memory, to holding it in memory, to retrieving it) and it is only recently that inactivation tools, such as inhibitory optogenetic molecules, could be turned on and off fast enough to distinguish between different phases. This project will use optogenetic inactivation in combination with this set of closely related working memory and decision-making tasks for the head-fixed rodent. The tasks are amenable to highly quantitative behavioral analysis. These features will allow a systematic and comprehensive quantitative probe of the causal contribution to working memory and decision-making of a very large set of regions across the dorsal cortex, deeper cortices, and targeted subcortical regions, including the cerebellum, the ventral tegmental area, the hippocampus, and the striatum. Other experiments will study the causal contributions of different genetically defined cell types, in targeted brain regions. Taken together, these experiments are expected to provide detailed information about how the interaction of particular neurons in the brain produces working memory and decisions, increasing knowledge about the brain basis of cognition.
Cortical Neural Coding & Dynamics
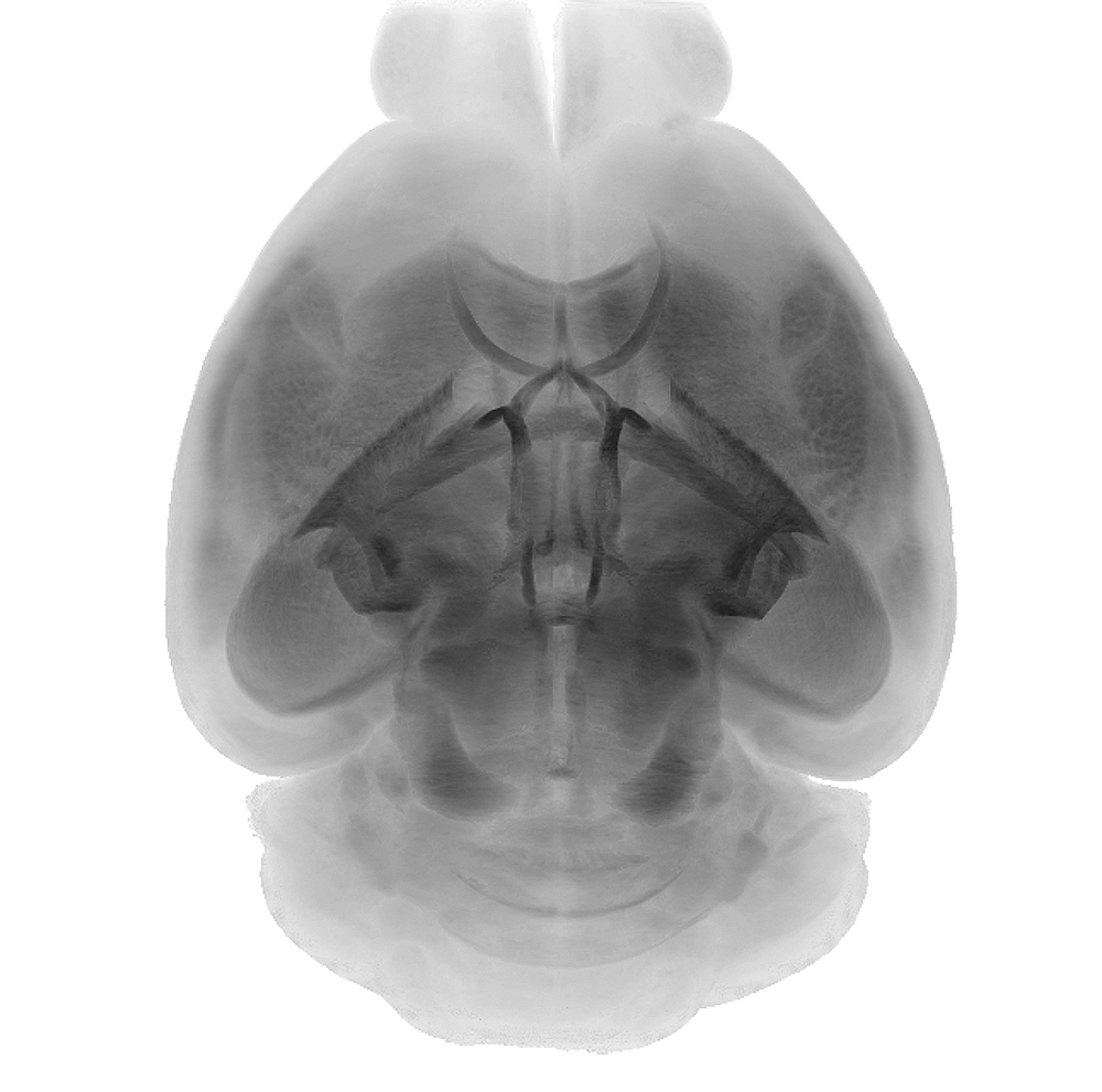
Cortical Neural Coding
& Dynamics
David Tank, Carlos Brody,
Ryan Cho, Mika Diamanti, Joshua Julian, Sue Ann Koay, Abigail Russo, Manuel Schottdorf
Working memory, the ability to temporarily hold multiple pieces of information in mind for manipulation, is central to virtually all cognitive abilities. This multi-component research project aims to comprehensively dissect the neural circuit mechanisms of this ability during a working memory and decision task based on accumulation of sensory evidence. Inactivation experiments in another component of the project will identify participating brain areas and their particular roles in such tasks. The goal of this project is to characterize the neural coding and dynamics in the neocortical brain areas found to have a causal role in the behavior. Initial inactivation results suggest that much of neocortex is involved. Thus, the project will produce a broad survey of neocortex using cellular-resolution calcium imaging with the most advanced optical-imaging technology, like the mesoscope. The results of this survey will be a dataset, unprecedented in the field of working memory and decision-making, that will greatly illuminate the nature of each region’s potential contributions and their computations. In parallel, neocortical dynamics will be measured with cell-type specificity, starting with populations of inhibitory neurons. Preliminary data shows choice-specific sequences and cue-locked cells in neocortical pyramidal neurons in six brain regions during an evidence-accumulation task involving navigation in virtual reality, but it is unclear whether these kinds of activity are specific to preliminary experiments or will generalize to diverse evidence-accumulation behaviors. To address this question, researchers will apply two-photon, cellular-resolution calcium imaging during other evidence-accumulation tasks, to explore the dependence on species (rat vs. mouse), behavioral readout (T-maze navigation vs. orienting vs. right/left licking), or sensory modality (towers, light flashes, airpuffs). The survey of neocortical activity at cellular resolution will be done one or a few areas at a time. Finally, the project will use widefield imaging fluorescence macroscopes, including a novel head-mounted version, for simultaneous imaging of all dorsal cortical areas during evidence-accumulation tasks. The maps will identify, for the first time, the simultaneously acquired spatial and temporal structure of region activation across the neocortical surface during an evidence-accumulation task in rodents. Based on the preliminary data, this work, along with the imaging results obtained in another project component, is expected to produce the most detailed information available to date on brain-wide activity, at cellular resolution, during performance of a cognitive task. Ultimately, these results will be used, in conjunction with perturbation and interaction data from other parts of the project, to develop and constrain biophysically realistic models of the neural mechanisms underlying working memory and decision-making across multiple brain areas.
Subcortical Neural Coding
& Dynamics
Ilana Witten, Sam Wang, David Tank
Scott Bolkan, Ryan Cho, Ben Engelhard, Edward Nieh, Marlies Oostland, Michael Siniscalchi
This project focuses on characterization of the neural dynamics underlying working memory and decision-making in a network of subcortical regions. Specifically, cellular-resolution two- and three-photon calcium imaging will be used to characterize neural coding and dynamics in dopamine neurons in the ventral tegmental area and substantia nigra, granule cells and Purkinje cells of the cerebellum, medium spiny neurons in the striatum, and pyramidal cells in the hippocampus. This data will be supplemented with multi-electrode recordings, which capture fast neural activity that calcium imaging cannot. The experiments will combine these two data types for the first time in the setting of a working memory and decision-making task. The results from this project, together with those from another component that investigates similar measures in neocortex, are expected to provide an unprecedented amount of data that will give new insight into the processing of task-relevant information during a cognitive task across a wide variety of cortical and subcortical areas. Another component will combine these results with temporally and spatially specific inactivation data from the other two components to build and constrain a biophysically realistic, multi-region computational model of the behavior.
Neuronal Interactions
Sebastian Seung, David Tank, Jonathan Pillow,
Mark Ioffe, Alexander Riordan, Adrian Wanner, Zhihao Zheng
Working memory, the ability to temporarily hold multiple pieces of information in mind for manipulation, is central to virtually all cognitive abilities. This multi-component research project aims to comprehensively dissect the neural circuit mechanisms of this ability across multiple brain areas. The overall goal of this project is to combine in-vivo functional imaging in mice performing working memory tasks in a virtual-reality setting with subsequent large-scale electron microscopy based circuit reconstruction. The reconstructed activity and connectivity patterns will be used to develop a neural circuit model of working memory and decision-making across multiple brain regions, constrained by neural activity, inactivation results, and connectivity.
Analysis & Modeling
Jonathan Pillow, Mark Goldman, Carlos Brody,
Avinash Avinash, Brian DePasquale, Ben Lankow, Rachel Lee, Zeinab Mohammadi, Iris Stone, David Zoltowski
Working memory, the ability to temporarily hold multiple pieces of information in mind for manipulation, is central to virtually all cognitive abilities. This multi-component research project aims to comprehensively dissect the neural circuit mechanisms of this ability across multiple brain areas. Large population recordings, such as those that will be obtained in other components of this proposal, open the door to assessing the dynamics of brain states on a single-trial, moment-by-moment basis. Yet their size and complexity present a challenge, as does the variety of data that will be collected, incorporating anatomy, behavior, neural activity, and perturbations. This project will develop and apply novel statistical analyses and modeling approaches to meet these challenges. The lion’s share of the variance in neural population activity is often dominated by variations in a small number of variables, which are called “latent variables.” This project will leverage the very large data sets, collected in other components of the project, of many simultaneously recorded neurons to develop advanced linear and nonlinear methods to identify the most informative latent variables. To analyze these datasets, the researchers will develop new latent variable discovery methods. First, they will combine advanced quantitative behavioral analysis with advanced statistical neural analysis. Second, they will combine latent space discovery with fitting of generalized linear models to neural data. The resulting nonlinear methods will provide an unprecedentedly complete statistical description of the data: these methods aim to simultaneously discover and capture the dynamics of the most important latent variables, and to produce a full statistical characterization of the responses of each individual recorded neuron. In biophysical modeling work, critical to creating a mechanistic understanding at the neural circuit level, this project will develop and test models of both local and multi-brain-region activity during working memory and decision-making. These models will build upon rigorous sensitivity-analysis techniques for identifying the critical network interactions underlying observed behavior. The models will be used both to interpret existing data and to design maximally informative experiments about inter-regional network interactions, and they will provide a principled platform from which to design future experiments that test specific hypotheses about function and further constrain the models.